Research Article
Rare-Earth Substituted Aqueous Ferro Fluid for Low Frequency Applications: An Easy Synthesis Route
1351
Views & Citations351
Likes & Shares
Water based Ferro fluids of appreciable gravitational and magnetic stability from sol-gel derived rare-earth substituted cobalt ferrite nanoparticles were prepared by an easy and fast synthesis route. Oleic acid has been used as the surfactant. Surfactant treatment has reduced the agglomeration of the particles to a greater extent and the particles in the fluid are showing super paramagnetic nature. Electrical characterizations have shown that the prepared magnetically controllable water-based Ferro fluid is a good candidate for low frequency applications due to high relative permittivity and low dielectric loss in the low frequency range. It is seen that rare-earth substitution in the proper concentration could fine tune the electrical properties for specific applications.
Keywords: Nanoparticles, Sol-gel preparation, Ferro fluid, Dielectrics
INTRODUCTION
In recent years, magnetic nanoparticles have evoked a strong enthusiasm among researchers due to its wide spectrum of applications like MR imaging, drug delivery cancer treatment catalysis [1-3] etc. Among the magnetic nanoparticles, cobalt ferrites with the formula CoFe2O4 have been extensively used in the areas of video and audio tapes, high density digital recording microwave absorbers [4,5] precursor for Ferro fluid [6] etc. The fascination with Ferro fluids which are suspensions of Nano sized magnetic particles in an appropriate carrier liquid arises from the fact that their flow and properties can be significantly altered by the influence of moderate magnetic fields. Agglomeration of the particles can be reduced by the use of suitable surfactants to result in a stable suspension. The partial substitutions of Fe3+ ions with rare-earth ions in the octahedral sites of spinel structure of ferrites have proven to manipulate the magnetic, structural and electrical properties [6-8].
EXPERIMENTAL
Gadolinium substituted cobalt ferrite nanoparticles of the formula CoGdxFe2-xO4 of appreciable homogeneity were synthesized using sol-gel auto-combustion method [9]. The nanoparticles thus obtained were sintered at around 150°C for nearly 20 hours in a muffle furnace to achieve uniform size distribution.
This powder was then ground well with few drops of oleic acid (AR grade, Merck) and added to de-ionized water at around 70°C and stirred for 30 min. The pH was adjusted until a suspension of magnetic nanoparticles was obtained. This suspension was centrifuged at an rpm of 1000 for 30 minutes and supernatant was decanted which resulted in a stable Ferro fluid. Same procedure was repeated for all the doping concentrations of gadolinium from x= 0.0 to 0.15 in steps of 0.05.
The morphology and particle size of the synthesized cobalt ferrite nanoparticles were estimated using Carl Zeiss FESEM and Jeol/JEM 2100 HRTEM. Crystallinity of the nanoparticles was analyzed using X- ray diffraction pattern recorded using Bruker AXS D8 Advance X- Ray powder diffract meter. M-H curve was recorded using Lakeshore VSM 7410 up to a maximum field of 25 KOe. Dielectric studies were conducted using 6500B Wayne Kerr impedance analyzer in the frequency range 100 Hz to 1 MHz and DC conductivity measurements using Keithley setup.
RESULTS AND DISCUSSION
From the FESEM image given in Figure 1a and HRTEM image in Figure 1b, it is evident that the prepared nanoparticles are of nearly spherical morphology. The histogram of particle size distribution given in Figure 1c shows that the sizes of majority of the particles fall in the range of 8-10 nm.
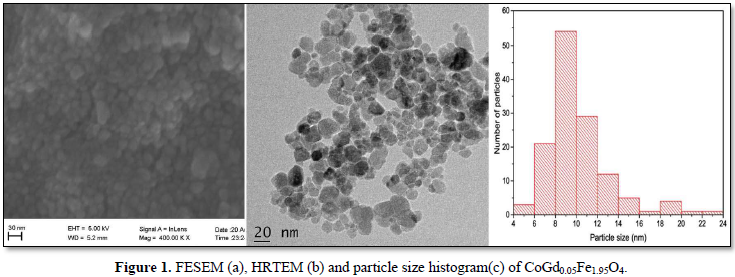
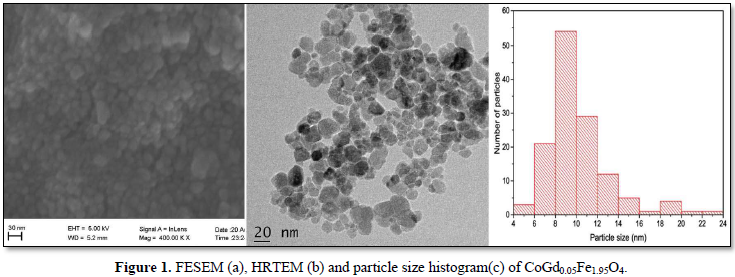
Crystallite size was estimated from the Hall-Williamson plots. Typical XRD pattern and Hall-Williamson plot obtained are given in (Figures 2a & 2b).
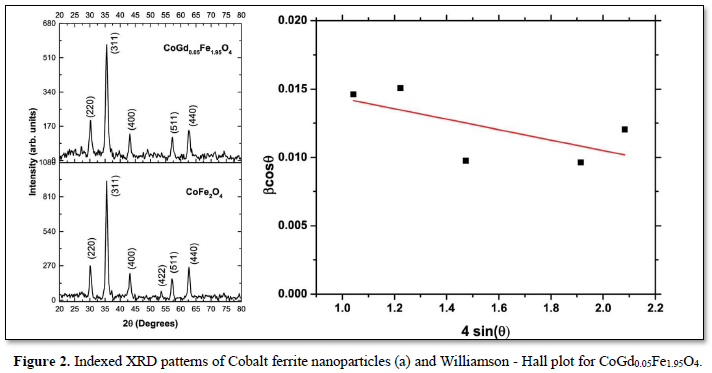
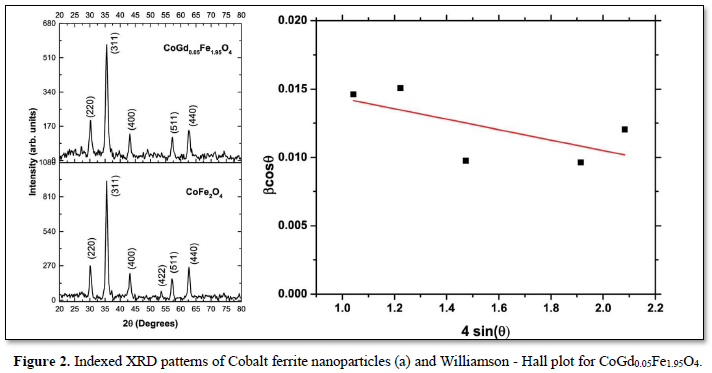
Using the Hall-Williamson method, the strain induced broadening of the XRD peaks is neglected and obtained much more accurate value of the estimated crystallite size. The crystallite size value calculated for CoGd0.05Fe1.95O4 is around 8.48± 0.06 nm.
The magnetic behavior is recorded at room temperature using a vibration sample magnetometer for the fluid with gadolinium concentration x = 0.05 which showed the best response to a neodymium strong magnet. The magnetic behavior is evident from the hysteresis curve given in Figure 3 which shows that the particles in the Ferro fluid are super paramagnetic in nature with zero coercivity and remnant magnetization while the particles in the powder form are ferromagnetic in nature. Paramagnetic behavior is exhibited when the size of the nanoparticles falls below the single domain size.
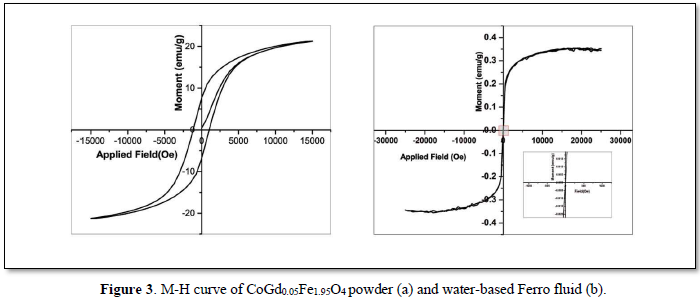
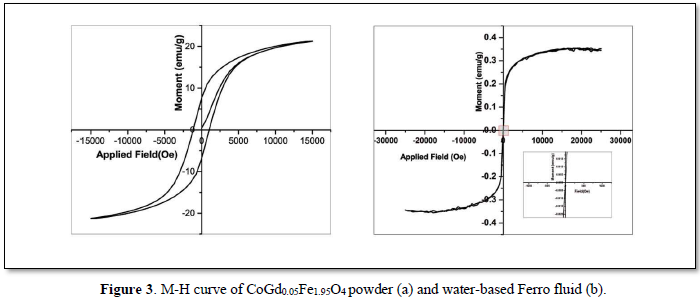
The electrical studies conducted for the Ferro fluids at room temperature of about 27°C are given in Figure 4. The relative permittivity values obtained for the different Ferro fluids in Figure 4(a) reveals that the magnitude of relative permittivity has increased to many folds compared to the base fluid at lower frequencies. The rapid decrease in relative permittivity at lower frequencies is explained by Koops theory [10] and interfacial polarization discussed in Maxwell- Wagner model [11]. When gadolinium is substituted, the Gd3+ ions replace the Fe3+ ions in the octahedral sites and hence decreases the number of Fe3+ ions which are responsible for the polarization. This causes the reduction in the relative permittivity. The conductivity decreases with increased doping concentration of gadolinium due to decrease in small polaron hopping as a result of replacement of Fe3+. The steady values of the relative permittivity and AC conductivity at high frequencies is due to the reason that the electron exchange between the ferrous and ferric ions fails to keep up with the alternating field at such high speeds. Hence the system shows reduced interfacial polarization causing constant relative permittivity and AC conductivity at high frequencies.
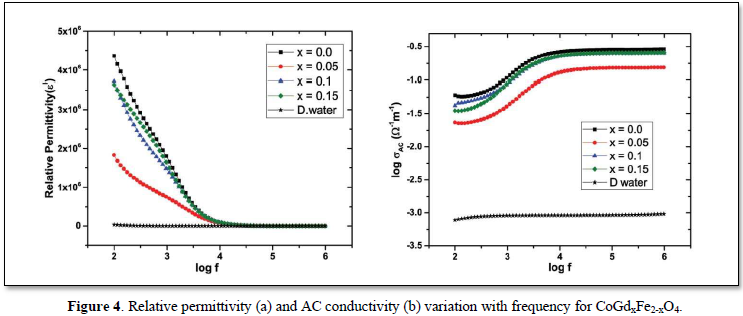
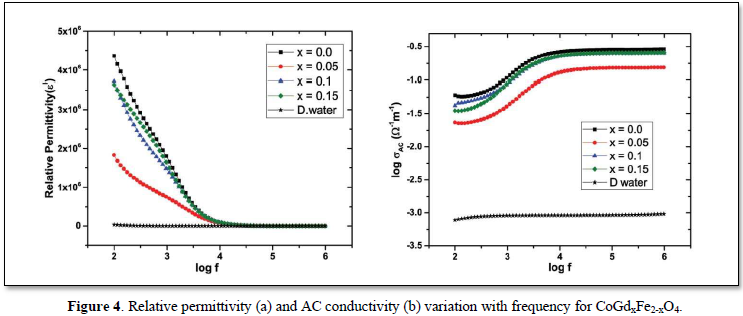
From Figure 5(a) it is clear that the Ferro fluids are showing very small dielectric loss at low frequencies compared to the base fluid as well as ferrite powder. The DC conductivity values given in Figure 5(b) are increasing with temperature similar to a semiconductor. The increase in conductivity with temperature may be due to the drift velocity enhancement of the thermally energized charge carriers and enhanced hopping of electrons. The fluids are showing appreciable decrease in the conductivity with increasing dopant concentration which may be due to the occupancy of Gd3+ ions in place of Fe3+ resulting in reduced conductivity. Further enhancement of conductivity for higher concentrations may be as a consequence of lattice distortions due to the comparatively larger ionic radius of Gd3+ ions.
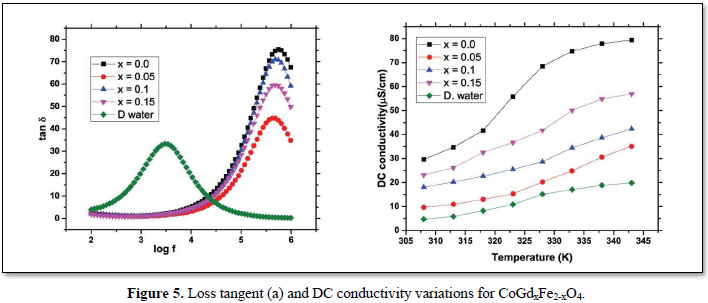
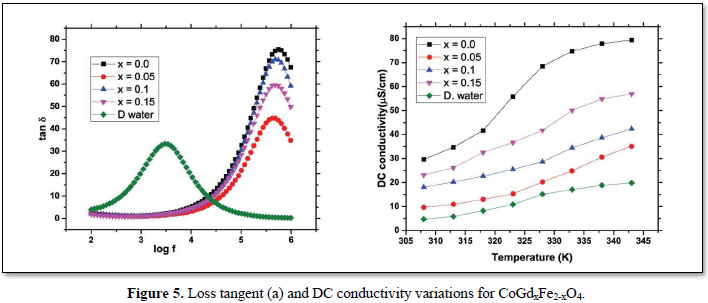
CONCLUSION
Magnetically controllable water-based Ferro fluids of appreciable magnetic and gravitational stability were prepared out of gadolinium substituted cobalt ferrite nanoparticles by means of a very easy, cost effective and fast synthesis route. Structural and morphological studies reveals that the particles are single crystalline and free from agglomeration which ensures high stability of the synthesized fluids. The particles in the Ferro fluid are well separated and its size falls below the single domain size showing super Para magnetism. The fluids could be potential candidates for low frequency applications due to their high relative permittivity and low dielectric loss. Fine tuning of the electrical properties could be achieved by means of rare-earth substitution at required concentrations for specific applications.
ACKNOWLEDGMENTS
Authors thank SAIF (Sophisticated Analytical Instrument Facility), Cochin for XRD and HRTEM analysis, Dept of Physics, CUSAT for FESEM analysis and SAIF, IIT Chennai for VSM measurements.Praveena M.G and E.M.Mohammed acknowledge the Kerala State Council for Science, Technology and Environment (KSCSTE), Kerala, India, for the financial support.
- Sun C, Lee JSH, Zhang M (2008) Magnetic nanoparticles in MR imaging and drug delivery, Adv Drug Deliv Rev 60(11): 1252-1265.
- Giustini AJ, Petryk AA, Cassim SM, Tate JA, Baker I, et al. (2010) Magnetic nanoparticle hyperthermia in cancer treatment. Nano Life 1(01n02): 17-32.
- Casbeer E, Sharma VK, Li X-Z (2012) Synthesis and photocatalytic activity of ferrites under visible light: A review. Sep Purif Technol 87: 1-14.
- Zi Z, Sun Y, Zhu X, Yang Z, Dai J, et al. (2009) Synthesis and magnetic properties of CoFe2O4 ferrite nanoparticles. J Magn Magn Mater 321(9): 1251-1255.
- Gul IH, Maqsood A (2008) Structural, magnetic and electrical properties of cobalt ferrites prepared by the sol-gel route. J Alloys Compd 465(1-2): 227-231.
- Kumar P, Sharma SK, Knobel M, Singh M (2010) Effect of La 3+ doping on the electric, dielectric and magnetic properties of cobalt ferrite processed by co-precipitation technique. J Alloys Compd 508(1): 115-118.
- Tahar BL (2008) Magnetic properties of CoFe 1.9 RE 0.1 O 4 nanoparticles (RE=La, Ce, Nd, Sm, Eu, Gd, Tb, Ho) prepared in polyol. J Magn Magn Mater 320(23): 3242-3250.
- Xavier S, Jacob BP, Thankachan S, Mary R, Mohammed EM (2013) Effect of Dy 3 + substitution on magnetic and electrical properties of nanocrystalline cobalt ferrite. J Nanosci pp: 1-12.
- Xavier S, Jiji MK, Thankachan S, Mohammed EM (2014) Effect of sintering temperature on the structural and electrical properties of cobalt ferrite nanoparticles. AIP Conf Proc 1576: 98-101.
- Koops CG (1951) On the Dispersion of Resistivity and Dielectric Constant of Some Semiconductors at Audiofrequencies. Phys Rev 83(1): 121-124.
- Wagner KW (1913) Dissipation of energy under AC. Ann Phys 40: 817-855.
QUICK LINKS
- SUBMIT MANUSCRIPT
- RECOMMEND THE JOURNAL
-
SUBSCRIBE FOR ALERTS
RELATED JOURNALS
- Journal of Oral Health and Dentistry (ISSN: 2638-499X)
- Journal of Otolaryngology and Neurotology Research(ISSN:2641-6956)
- Journal of Nursing and Occupational Health (ISSN: 2640-0845)
- Journal of Psychiatry and Psychology Research (ISSN:2640-6136)
- International Journal of Diabetes (ISSN: 2644-3031)
- Journal of Pathology and Toxicology Research
- Journal of Carcinogenesis and Mutagenesis Research (ISSN: 2643-0541)